Microfluidics
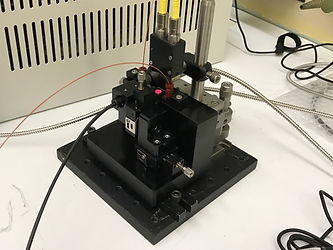

Set ups used in these studies, provided by Spectra Solutions
Microfluidics has emerged as a transformative technology for chemical processing, offering precise control over reactions while minimizing waste and enhancing safety—particularly in hazardous applications like nuclear fuel reprocessing. A critical advancement in this field is the integration of micro-Raman spectroscopy, which enables real-time, in situ monitoring of chemical species within microfluidic channels. The development of specialized Raman probes with micron-scale resolution allows researchers to interrogate small volumes with high accuracy, overcoming traditional limitations of bulk analysis. These systems are especially valuable for studying complex mixtures, where overlapping spectral bands or interfering species would otherwise complicate quantification.

Figure 1. Top left: Photo of the micro-Raman probe. Top right: Laser spot size (70 μm) on a USAF target. Bottom left: Probe focused on a microfluidic device. Bottom right: On-board camera view showing laser alignment within the microchannel.
One key study (Anal. Chem. 2018, 90, 2548) highlights the use of a novel micro-Raman probe with a 70 μm laser spot size to monitor HNO₃ and NaNO₃ in microfluidic devices. By employing chemometric modeling (e.g., partial least-squares regression), the researchers accurately quantified species despite spectral overlaps, demonstrating the probe’s ability to handle confounding variables like baseline shifts and matrix effects. A follow-up study (Analyst 2018, 143, 1188) compared macro- and micro-Raman probes, showing that the latter could effectively measure HNO₃ concentrations in microliter volumes, even when calibration data came from larger path-length samples. This work underscored the flexibility of micro-Raman systems for scaling down laboratory processes without sacrificing accuracy.
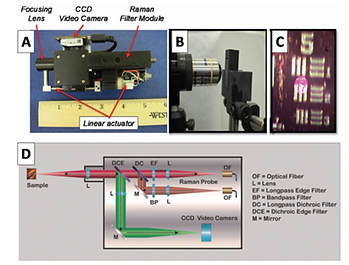
Figure 1. A/B: Micro-Raman probe positioned in front of an 8 μL flow cell. C: Laser spot (70 μm diameter) imaged on a USAF target. D: Schematic of the probe’s optical path, including the dichroic filter and CCD camera.
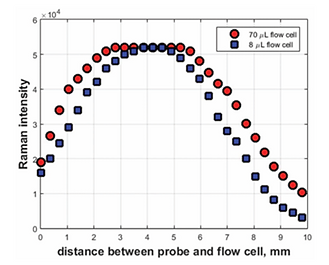
Figure 4. Nitrate band intensity vs. probe-to-flow-cell distance, showing optimal positioning for signal collection.
Further advancements were demonstrated in a 2021 study (Anal. Chem. 2021, 93, 1643), which combined Raman and UV-vis spectroscopy to monitor biphasic extractions of Nd(NO₃)₃ in microfluidic devices. The system tracked both organic and aqueous phases in real time, using chemometric models to correlate spectral data with concentration changes. The results aligned with macroscale extraction kinetics, validating microfluidics as a reliable platform for process optimization. Another study (Anal. Chem. 2018, 90, 8345) focused on interfacial mass transfer in HNO₃-TBP extraction, using Raman spectroscopy to measure kinetic rates within microchannels. The probe’s ability to capture transient species and dynamic concentration profiles highlighted its potential for studying fast chemical processes in confined environments.
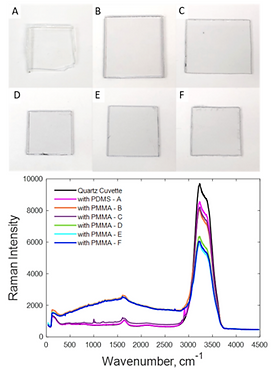
Figure 1. Raman spectra of water measured through different chip materials (quartz, PMMA, PDMS), emphasizing quartz’s minimal interference.

Figure 4. A: Schematic of alternating aqueous/organic droplet flow in the microchip. B: "Pinhole" camera image of droplet formation in the microchannel.
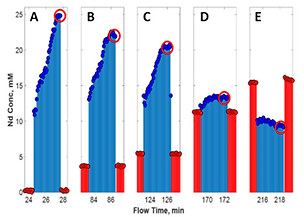
Figure 7. Time-resolved concentration profiles of Nd³⁺ in aqueous/organic phases, showing extraction kinetics.
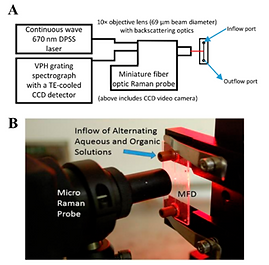
Figure 1. A: Schematic of the micro-Raman system (670 nm laser, spectrograph, CCD detector). B: Photo of the probe focused into the microfluidic channel.
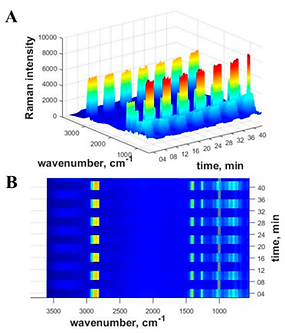
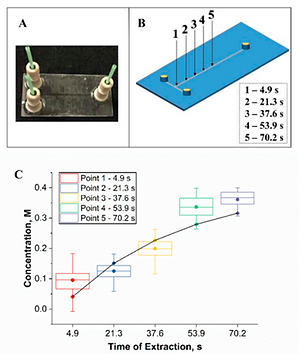
Figure 4. A: 3D plot of Raman intensity vs. wavenumber vs. time, showing alternating aqueous (NO₃⁻ peak) and organic (C–H stretch) phases. B: Overhead heatmap view of the same data, highlighting droplet segmentation.
Figure 6. A: Microfluidic T-junction design for biphasic flow. B: Probe measurement positions along the channel for kinetic studies. C: HNO₃ concentration vs. time plot, showing interfacial mass transfer rates.
These studies illustrate how micro-Raman spectroscopy—paired with multivariate analysis—enables precise, real-time monitoring in microfluidic systems. The technology bridges the gap between lab-scale experiments and industrial applications, offering a path toward safer, more efficient chemical processing. As microfluidics continues to evolve, Raman-based monitoring will likely play an increasingly vital role in advancing chemical engineering and nuclear science.
Publications:
Amanda M. Lines, Gilbert L. Nelson, Amanda J. Casella, Job M. Bello, Sue B. Clark, and Samuel A. Bryan, "Multivariate Analysis To Quantify Species in the Presence of Direct Interferents: Micro-Raman Analysis of HNO3 in Microfluidic Devices," Analytical Chemistry 2018 90 (4), 2548-2554
Gilbert L. Nelson, Amanda M. Lines, Amanda J. Casella, Job M. Bello and Samuel A. Bryan, "Development and testing of a novel micro-Raman probe and application of calibration method for the quantitative analysis of microfluidic nitric acid streams," Analyst, 2018, 143, 1188
Gilbert L. Nelson, Susan E. Asmussen, Amanda M. Lines, Amanda J. Casella, Danny R. Bottenus, Sue B. Clark, and Samuel A. Bryan, "Micro-Raman Technology to Interrogate Two-Phase Extraction on a Microfluidic Device," Analytical Chemistry 2018 90 (14), 8345-8353
Gilbert L. Nelson, Hope E. Lackey, Job M. Bello, Heather M. Felmy, Hannah B. Bryan, Fabrice Lamadie, Samuel A. Bryan, and Amanda M. Lines, "Enabling Microscale Processing: Combined Raman and Absorbance Spectroscopy for Microfluidic On-Line Monitoring," Analytical Chemistry 2021 93 (3), 1643-1651